Small Modular Reactors are needed in African countries – Watts Up With That?
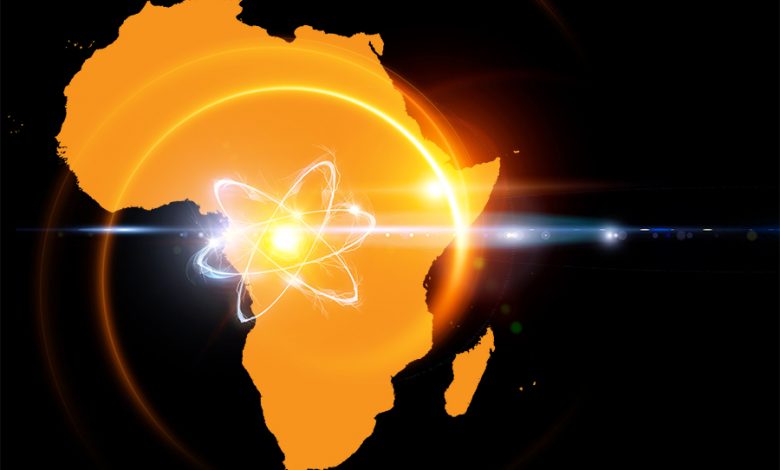
Dr. Kelvin Kemm
Nuclear power is not new. It has been in operation for over half a century, with an excellent record for providing reliable baseload electricity.
Not only has the electricity turned out to be reliable, but it is also the safest, greenest and most inexpensive electricity currently available to mankind. The proof exists.
But to judge from much of the barrage of anti-nuclear propaganda, which has been projected by anti-nuclear activists, people can be forgiven for doubting such claims. Let us consider the infamous Fukushima nuclear power incident in Japan. Not one single person was killed or injured by nuclear radiation at Fukushima. Read that again. Not one single person!
Nuclear power stations run for very many years. Over their lifetime the electricity produced is inexpensive. The concept of ‘cost’ can be judged only over a life-cycle, not at initiation.
Ask whether a brand new Boeing or Airbus passenger aircraft cost a lot of money. It is wrong to look at the purchase price on the day of delivery You need to examine the situation at the end of the life of the aircraft, when you can then count the total passengers carried, tally operating costs, and then work out its profitability to the air carrier, and hence the actual operational ‘cost’. Don’t confuse the concepts of ‘purchase price’ and ‘cost’. They are very different.
Anti-nuclear activists go out of their way to induce public confusion with respect to this important distinction.
Nuclear power is highly profitable. Both airlines and nuclear power operators know how to calculate these cost figures very accurately. That is why both are confident in making a profit on a new investment in their respective fields of expertise.

Over the past half-century, nuclear power has proven itself to have been highly reliable and profitable. It will be even more reliable and more profitable in the future.
Many people do not seem to realise that nuclear technology has advanced dramatically during recent decades. Modern nuclear reactors are far more advanced now than ever before. Modern fabrication techniques, modern electronic systems, modern metal alloys, modern computer control, modern internet-based operating surveillance, modern robotics; the list goes on. All this means we now have ‘space-age’ nuclear reactors, compared to those built in the previous century.
As nuclear reactors developed over the first half-century of nuclear power, they became larger, and all were based on having a large body of water available, such as an ocean or major lake.
But this design concept had a built-in self-limiting barrier. They needed the water! However, many countries do not have a coastline. Many don’t even have major lakes or rivers. So the customer-base was limited.
Big and small nuclear
Africa is enormous. It is larger than the US, Europe, China, India and Japan added together. That means even some African countries which do have an ocean coast also have major areas very far from the ocean that need to be electrified. South Africa is a good example.
South Africa is the same size as the whole of Western Europe. The distance from South Africa’s inland capital, Pretoria, to Cape Town is the same as the distance from Rome to London. The world’s most southerly nuclear power station, Koeberg, is situated on the southern coast of South Africa, from where it serves the southern regions.
But major mining areas are 1000 km inland, in arid areas with no large water bodies, while South Africa’s vast coal deposits and coal-fired power stations are clustered in the far north east of the country. That means very long transmission lines are required to serve the country.
The nuclear power from Koeberg is South Africa’s cheapest and most reliable electricity.
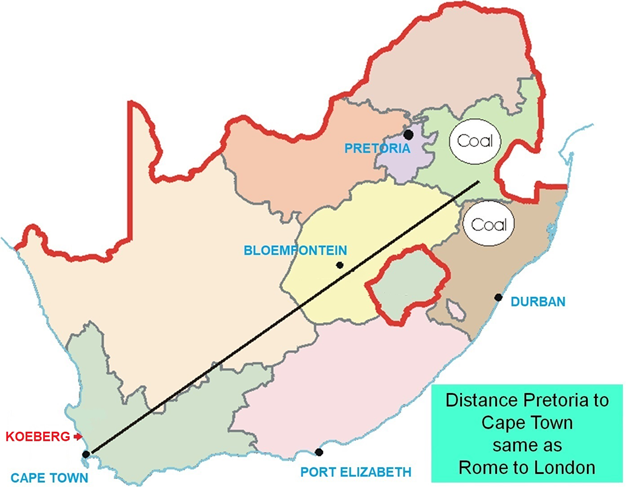
Note that the length of the black line is the same as the distance from Rome to London
So during the last decade of the 20th Century, South African nuclear technologists decided to investigate building a small nuclear reactor which would not need water cooling. Its purpose was to serve large mining and industrial complexes, and arid inland regions.
There certainly is still a need for large nuclear power plants, but there is also a desperate need for much smaller ones too.
Initial design criteria for a new small reactor were that it should be easy to build, and easy to add more reactors to an existing complex. The idea of pursuing a ‘modular’ concept became a cornerstone of design. Another very important design constraint was that the electricity produced should not be more expensive than the current selling price of South Africa’s coal-fired electricity.
The Small Modular Reactor (SMR) had to be gas-cooled, so inert helium gas was chosen. Fuel had to be easy to load into the reactor, and easy to transport, so graphite-based fuel balls the size of cricket balls were chosen. The term ‘pebbles’ was adopted for the fuel.
Thus was born the South African Pebble Bed Modular Reactor (PBMR) Project.
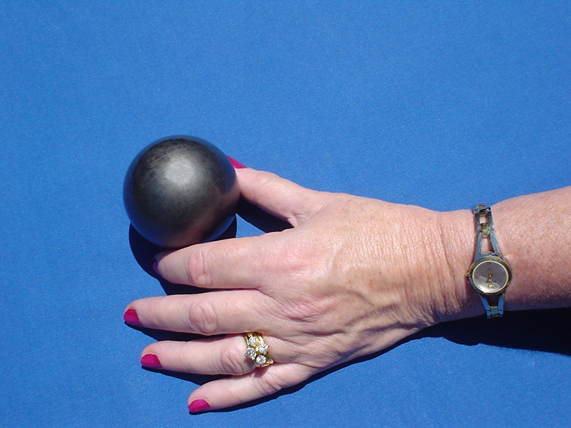
The fuel was fabricated near Pretoria.
South African SMR development
This project developed steadily to the point at which the total staff complement was some 2 000 people. The Project advanced such that by 2008 the PBMR team was ready to construct the First Of A Kind (FOAK) prototype. The site had been selected and approved and all was ready for the starter’s pistol to fire.
Then fate intervened. The world 2008 financial crisis was initiated in the US, as a result of a foolish housing mortgage policy. This cascaded around the world. Big international banks which had become involved in the financing deals for the PBMR suddenly became bankrupt or unable to honour their pledges to the PBMR. At the same time South Africa had an unexpected change in government, with a new president and cabinet taking office. A number of major projects were then put on hold, the PBMR are being one of them. Nuclear technologists thought this ‘hold’ would last for a few months, but in fact it turned out to be years.
Further delay was induced due to the extreme green sentiment which started to rage around the World, with a prime target of the activists being energy. The targets included fossil fuels, nuclear power and even hydroelectric. Romantic visions of the world running on solar and wind power became the darling image for much of the media. The realities of physics and technological engineering were largely overlooked or ignored. Sound reasoning became a victim of the circumstances, as strange images appeared, such as a schoolgirl lecturing the United Nations on world energy policy.
A group of far-sighted SMR developers in Pretoria then formed a private company to develop a variant of the PBMR. It was financed by a cluster of companies and individuals who believed in the SMR concept.
Some careful review planning was done and important decisions taken. Primary among them were decisions to produce a small nuclear power plant which was inexpensive to design and build, and could be built rapidly.
This led to significant design decisions. The PBMR had been designed with a Brayton cooling system in which helium travels through the reactor core and then directly into the turbine; it then returns to the reactor. The reactor outlet temperature of the Helium was 940°C. This was an excellent design.
The HTMR-100 design
The new team embarked on a new SMR variant, named the HTMR-100. However two significant changes were introduced. The team decided to reduce the outlet gas temperature to 750°C, and pass the helium into a conventional water heat-exchanger, and not directly into the turbines, which meant the turbines would run on steam.
These were very important decisions for the ease of development, and for the consequent economics. The lower temperature reduced a great deal of complexity, with respect to the design and fabrication of the reactor.
Inserting a water heat-exchanger meant the turbines would be driven using conventional steam, and not helium. In turn this meant that everything ‘downline’ of the reactor itself could be purchased off-the-shelf, and therefore did not induce any financial uncertainty into these aspects of the design, or into the construction costs of all that portion of a power station. All this is standard equipment and is well understood in the engineering world.
Bearing in mind the ‘modular’ imperative in the SMR concept, this approach of the HTMR-100 is ideal for deployment into other African countries, and also anywhere where an identified construction site could be a challenge in terms of distance to transport and integrating large sub-assemblies.
The design and development of the HTMR-100 is far advanced, having been developed for over a decade.

Scale is 200m across the x-axis in the picture
The model shown in Figure 4 is the current 100-megawatt nuclear power station, built to scale from the engineering drawings. This model is not a futuristic concept; it is a real design that currently exists. The model shows placement in an arid area, in a typical South African mining town setting. There is no large body of water available, and since water is generally scarce in such environments, no extensive manicured gardens. This is the reality of a workhorse small nuclear power station in Africa.
The HTMR-100 is a Generation IV (Gen IV) reactor, which is a high-temperature gas-cooled reactor. It is a significant advance on the much lower temperature water-cooled reactors. The advanced technology that has been achieved is partly a result of the initial extensive design and development of the PBMR.
A massive amount of work went into the PBMR development, over a period of more than a decade. Not only was advanced science and engineering addressed, but also all the operational and safety procedures, system qualifications, and all the other paper-based nuclear processes that are so important to the functioning of a modern nuclear power station.

Few people realize that the PBMR project advanced as far as building demonstration systems, which all worked exactly as designed. In 2008 the PBMR team was ready to start construction.
Process heat applications
The PBMR was designed to produce electricity, but it did not take long to realise that the high temperature of the outlet gas offered significant potential applications for using the process heat, without needing to produce electricity first. So the HTMR-100 was designed to employ its 750°C outlet gas in a process-heat function, as well as producing electricity.
This means a high-temperature gas reactor has many more potential applications than conventional low-temperature reactors.
These range from mining frozen tar-sands in high-latitude Canada, to extract oil; to converting coal to petrol and diesel, as is done in South Africa. Many more process-heat applications will be developed, as high-temperature SMRs are deployed.
During the last couple years there has been a rapid worldwide increase in interest in the potential of nuclear power supplied by SMRs. Now we find that it is not only nuclear scientists and engineers who recognize the potential of Small Modular Reactors, but also politicians and business leaders.
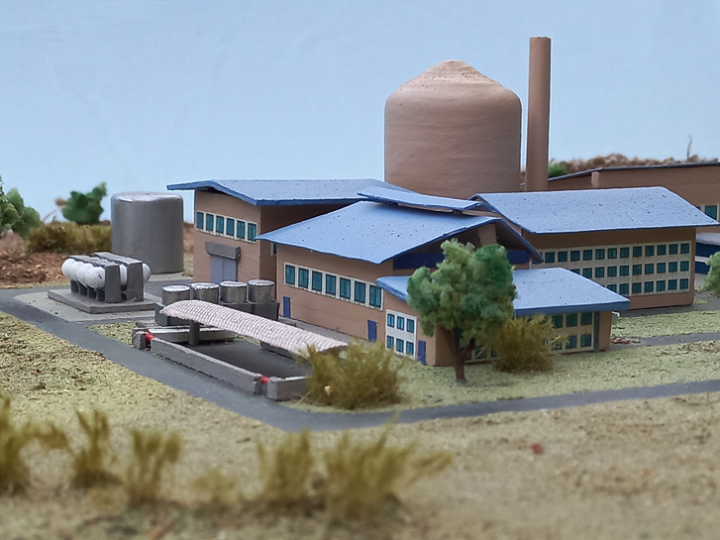
Current world attitude
For decades internationally, an unreasonable anti-nuclear attitude has prevailed amongst many people. Part of this was a legacy of the nuclear bombings in Japan which finally ended World War II. Large portions of the international public were led to believe that a nuclear reactor could explode like a bomb. It can’t. So in many cases the public linked a ‘no nukes’ sentiment to weapons and nuclear reactors. This was very unfortunate, but slowly as decades passed the thinking sections of the public realised that reactors were not nuclear explosives.
But sadly some anti-nuclear political activists have tried to keep false nuclear reactor narratives alive. They also propagated the false story that nuclear radiation itself is so dangerous that even some minute amount is somehow deadly, or will lead to terrible long-term effects. Such assertions are far from the truth.
Thankfully, over the past two years the worldwide attitude toward nuclear power generally, and SMRs particularly, has been changing significantly in a positive manner.
Anti-nuclear activists still try to oppose the acceptance of nuclear power, but wisdom and an appreciation of reality have started to take hold among many people.
A contributing factor concerning negative sentiments about fossil fuels and carbon dioxide emissions has played a role in causing people in authority to declare that nuclear power emits no CO2 and is therefore accepted as ‘green’ in the context of the CO2 emissions argument. Operating reality has shown that romantic ideas about wind and solar power running countries are just not feasible.
Over the past two years there has been a rapid acceptance of the critical worldwide potential role of SMRs, in any society.
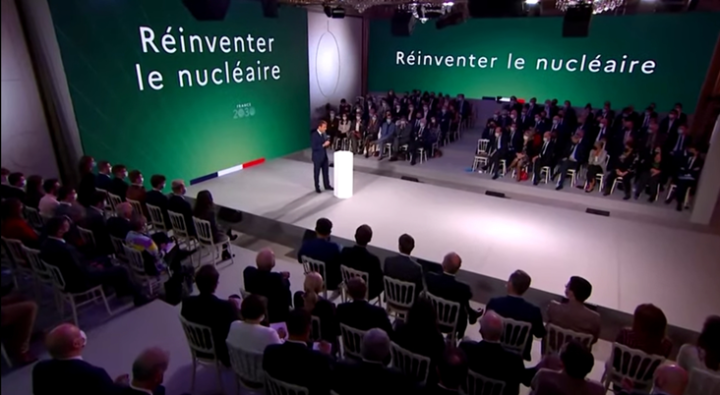
Previously, nuclear power was generally imagined to be large nuclear plants, which were the exclusive domain of a few wealthy, technologically advanced countries. Now with the SMR development advancing so rapidly, politicians and voters have realised that an SMR can be deployed in most countries of the world, should any country want one.
SMRs can be placed virtually anywhere they are needed, by any country. They can even be owned by private companies.
Types of SMR
The realisation of the extreme value of an SMR system has spawned significant international interest, and activity.
The International Atomic Energy Agency (IAEA) has defined SMRs as being 100 MW to 300 MW in output, in contrast to conventional large nuclear plants which can be of the order of 2000 MW to 4000 MW. That makes SMRs a completely different philosophy, compared to the past public understanding of nuclear power.
The IAEA also published a handbook listing many types of SMR currently being conceptualised or developed around the world. They range in nature considerably.
For example, in a molten salt design the uranium fuel is introduced into a salt that becomes liquid at a higher temperature, and then flows through the reactor.
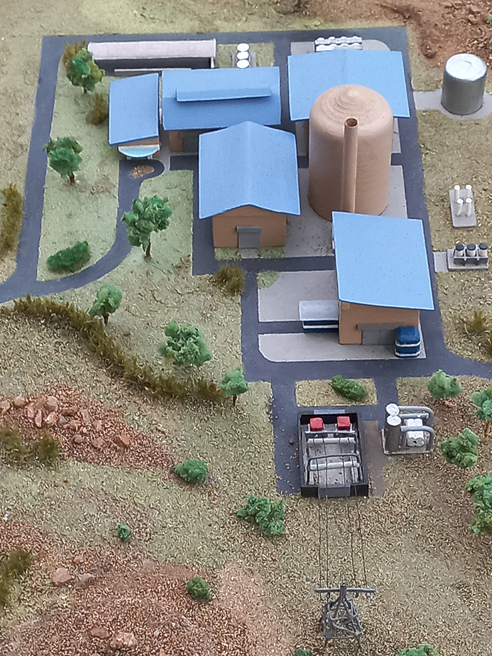
The HTMR-100 uses ‘pebbles,’ which are graphite balls containing small grains of Uranium that in turn are coated with a thin protective shield of silicon carbide. That makes the Pebble fuel itself exceptionally safe from any leaks of radioactive particles, let alone the protective containment of the entire reactor itself.
The fuel balls are easily transported by road over long distances, making them ideal for African conditions. They are very robust and can withstand rough handling, in contrast to the fragile large metal fuel assemblies used in large nuclear reactors.
Taking an overall view, the HTMR-100 is an ideal nuclear reactor system to be deployed in any area, including arid and remote regions.
A significant quantity of fuel can be safely stockpiled in underground bunkers, so supplies covering months or years can be kept on site. Even if road or rail links are disrupted, reactor operation will not be affected.
Africans are proud that the Pebble Bed design and fuel were developed in South Africa, a pilot plant was built and fuel of an extremely high standard was produced. International tests verified all this.

Current development position
A nuclear development team is currently working on SMR development in South Africa. Unfortunately the team is small and very underfunded. There is no government funding. A significant number of nuclear professionals is available to rapidly expand the team if funds become available.
The development group is open to international funding. Being a private company, the HTMR-100 group is in a position to entertain a wide range of proposals. A number of approaches from interested parties have already been made, as the international favourable attitude toward SMR development advances.
Fortunately South Africa has an energy minister who appreciates the need and reality of providing a reliable supply of baseload electricity to the country. He has launched a program to build 2,500 megawatts of new nuclear power, to add to the existing nuclear power base, and has specifically stated his support for including SMRs.
So the government attitude towards SMR development and deployment in South Africa is positive. In addition, about a dozen other African countries have declared their intention of following a nuclear power path in the future. Some have already set up national nuclear bodies to advance their plans.
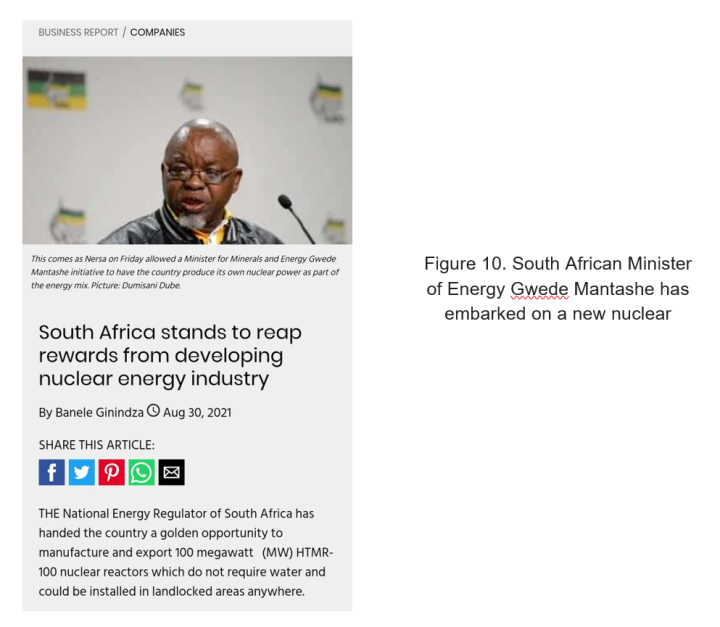
Clearly the potential exists for a network of SMRs in Africa. These can be in a number of African countries, but operating in collaboration such that operating experience, maintenance functions and general management experiences are shared for mutual benefit.
The creation of such an African network of SMR operators seems inevitable.
Industrial fabrication site – Kragbron
In South Africa a nuclear site for the construction of a prototype SMR nuclear reactor has been identified at the Pelindaba site of the South African Nuclear Energy Corporation (Necsa), near Pretoria. It is close to where the HTMR-100 team is currently working.
In addition, another site in the Free State Province has been offered as a site for setting up facilities for fabricating multiple reactor components and assemblies, for national and international deployment.
This is the town of Kragbron, which is very close to the major industrial fabrication area of Vanderbijlpark, where many facilities provide heavy engineering, and thus not only expertise and experience for modern fabrication, but also industrial users for the electricity.
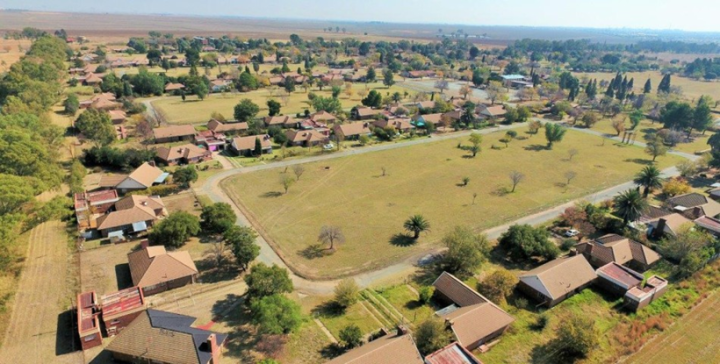
Suitable industrial buildings and an infrastructure also exist at Kragbron.
In fact the owners have already said that they would also like to build an operating commercial HTMR-100 there as soon as possible, to supply additional reliable electricity to the region.
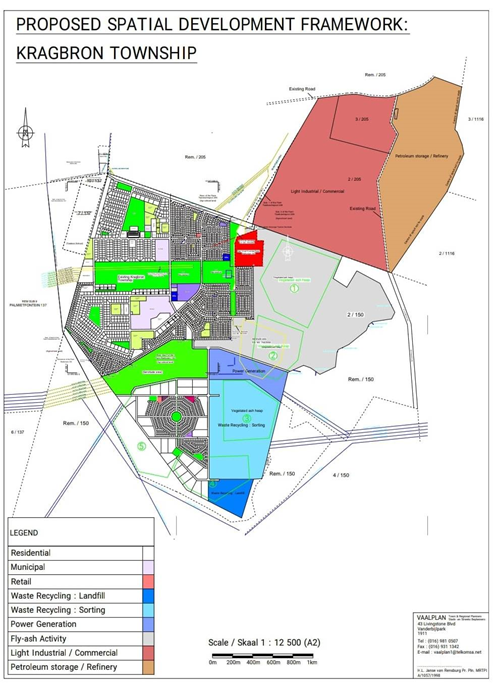
The town of Kragbron is privately owned and available for any other related technology developments, so an entire fabrication industry could be built there for any applicable technology developments.
Small grids
The countries of Europe have such an interconnected electricity grid system that each one acts as a backup for the others. For example, when the UK is short of electricity it imports nuclear-based electricity from France and hydro-power from Norway, via undersea cables.
Within continental Europe, electricity trading across borders happens constantly, each country backing up its neighbours. In Africa this is not the case; trans-border electricity movement is minimal. African countries are so large that even within a single country moving electricity from one region to another can be a major challenge, requiring transmission cables hundreds of kilometers long.
However a huge advantage of an HTMR-100 is that the reactor can be placed anywhere. Furthermore, a single reactor can have it own electricity grid, not necessarily connected to the rest of the country. Thus, for example, a remote mining community could have one or two reactors serving its own dedicated grid which is only 10 or 20 km wide. This can then be integrated into a larger grid later when the authorities wish to do so.
Small nuclear reactors offer extensive flexibility, and they run continuously, independent of day or night, rain or sunshine, wind or no wind. They also do not need a system for delivering a continuous fuel supply; deliveries two or three times a year would be sufficient.
Nuclear power is the future. Within the near future, there will also be nuclear reactors on the moon and Mars. They almost certainly will be based on a gas-cooled SMR principle.
______________
Dr Kelvin Kemm is a nuclear physicist and CEO of Stratek Business Strategy Consultants, a project management company based in Pretoria. He is part of the Stratek Nuclear Consortium, which is developing the HTMR-100 nuclear reactor. He carries out business strategy development and project planning in a wide variety of fields.